contents
- SIRH7/LDOC1
(1) The proper structure allows for precise timing
(2) Placental P4 production in rodents - SIRH11/ZCCCH16
(1) Sirh11/Zcchc16 KO mice exhibit behavioral abnormalites
(2) Diversification of eutherian lineages by variations in SIRH11/ZCCHC16 - Why are SIRH/RTL genes enriched on the X chromosome?
- Mammalian evolution by genes exapted from LTR retrotransposons and retroviruses
- What was the selective pressure on emergence of viviparity in mammals?: a relationship to oxygen concentration in the atmosphere
1. SIRH7/LDOC1
(1) The proper structure allows for precise timing
In mice, Sirh7/Ldoc1 plays an essential role in controlling the differentiation and maturation of a wide variety of placental cells, thereby regulating both the amount and timing of placental hormones37. The placenta is a major endocrine organ during gestation: giant trophoblast cells produce progesterone (P4) and placental lactogen I/II (PL1 and 2), and spongiotrophoblast and cytotrophoblast cells produce several prolactin-like proteins in order to maintain pregnancy and determine the timing of parturition.
Sirh7/Ldoc1KO placentas exhibit a perturbed structure in the three layers because of abnormal differentiation/maturation of most of the placental cells (Fig. 1, left) and overproduction of P4 in the mid gestation stage leading to delayed parturition in pregnant mice for one or two days due to the remaining serum P4 concentration on d18.5, the day before delivery (Fig. 1, right). The average number of their weaned pups was significantly lower than the controls (1.7 and 5.8 pups per litter, respectively) because of poor maternal care. As reproductive success is one of most important selection factors in the Darwinian theory of evolution, SIRH7/LDOC1 may have been positively selected because of the reproductive advantage it conferred, and thus was conserved in the eutherian reproduction system.
The deletion of Sirh7/Ldoc1 led to placental structural abnormalities, and at the same time to the endocrinological abnormalities, such as the overproduction of placental P4, resulting in delayed parturition associated with a low pup weaning rate. This means that the healthy placental structure and correct composition of a variety of placental cells determine the exact time of parturition via complex endocrine regulation. “Proper structure allows for precise timing”, which may be a fundamental rule of biology.
(2) Placental P4 production in rodents
P4 plays an essential role in the protection of the conceptus from maternal immunity, as ovariectomy at any time during the course of pregnancy causes rapid abortion that can be rescued completely by intraperitoneal P4 injection. In humans, P4 is first produced in the ovary and then in the placenta after 8 weeks of gestation, whereas in rodents the ovary has long been thought to be the only P4 productive organ throughout the course of pregnancy1. It is known that the serum P4 concentration exhibits temporal downregulation around d10.52 (Fig. 1, right), presumably because the ovary stops P4 production intrinsically on d10.5, as observed in pseudopregnant mice . Therefore, it has long been a question how embryos overcome the low serum P4 level during the period d9.5-10.5. We propose that placental P4 compensates for the reduced serum P4 concentration in the pregnant mice in a site-specific manner or contributes to maintain the minimum serum P4 level. The next question would be what kind of placenta signal(s) would be essential to recover ovarian P4 production for the last half of pregnancy.
In the course of this study, we developed an improved P4 assay method by using mass spectrography37. This enables not only the direct identification of the organ producing P4, but also determination of the timing and levels during the course of pregnancy. In fact, we successfully demonstrated the presence of mouse placental P4 in mid-gestation when a temporal reduction of serum P4 level occurs due to a shift from the corpus luteum of pseudopregnancy to pregnancy. We believe that this method opens a new chapter in reproductive biology, not only in eutherians but also in marsupials, as well as the reproduction in oviparous monotremes and non-mammalian vertebrates, such as birds, reptiles and amphibians. We assume that placental P4 production is commonly observed in at least eutherians, such as humans, in a constant manner, and transiently in mice.
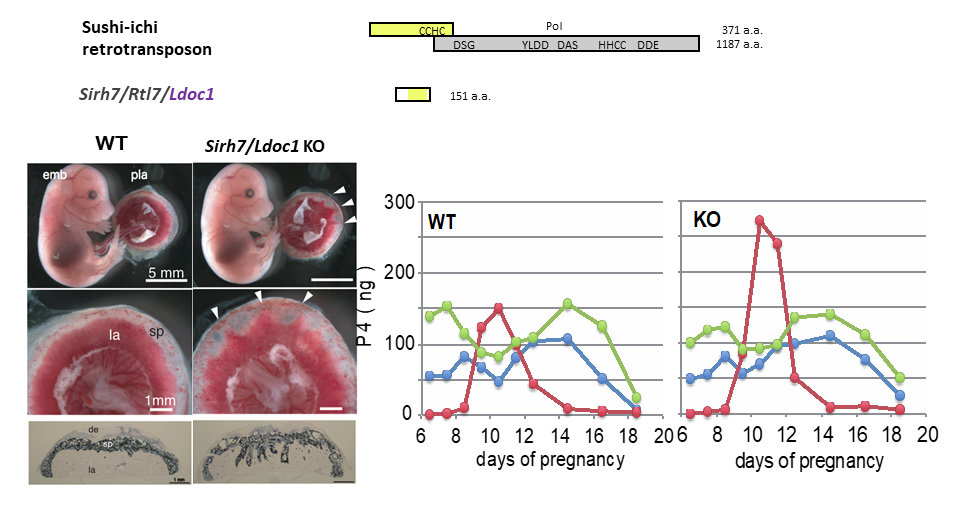
(Left) Fetuses and placentas on d15.5. The arrowheads indicate the regions where the labyrinth layers almost reached the maternal decidua. Despite the placental abnormalities, the Sirh7/Ldoc1 KO fetuses had a normal appearance and were born normally. (Right) Placental P4 production was observed mid-gestation when ovarian P4 production was temporally reduced, suggesting the importance of placental P4 production in mice. Overproduction of placental P4 was observed in Sirh7/Ldoc1 KO mice, leading to delayed parturition of pregnant females associated with a low pup weaning rate.
Original reference (Theme 4, reference 37)
- Naruse M et al. Sirh7/Ldoc1 knockout mice exhibit placental P4 overproduction and delayed parturition. Development. 141, 4763-4771 (2014).
References
- Malassine A, Frendo J-L and Evain-Brion D. A comparison of placental development and endocrine functions between the human and mouse model. Hum Reprod Update 9, 531-539 (2003).
- Virgo B B and Bellward G D. Serum progesterone levels in the pregnant and postpartum laboratory mouse. Endocrinol 95, 1486-1490 (1974).
2. SIRH11/ZCCCH16
(1) Sirh11/Zcchc16 KO mice exhibit behavioral abnormalites
Among the SIRH/RTL genes, Sirh11/Zcchc16/Rtl4 is unique because it does not exhibit any placental expression during mouse development, but is constantly expressed high in the brain38. Sirh11/Zcchc16 KO mice do not exhibit any lethality nor growth abnormalities, but do exhibit abnormal behaviors related to cognition, including attention, impulsivity and working memory38.
We first noticed that Sirh11/Zcchc16 KO mice do not adjust to routine processes because they always displayed agitated movement in their cages when staff personnel entered the breeding room, and sometimes jumped out when their cages were exchanged, even after a long breeding period. Comprehensive behavior tests clearly confirmed this behavioral abnormality. In the home-cage activity test for 5 continuous days, they always exhibited significantly higher activity during the dark period, especially just after the “light to dark” as well as just before the “ dark to light” transition periods, while normal control mice adjusted gradually to lower levels (Fig. 2A). In the Y-maze test, they had a poor working memory. Most impressively, some of the KO mice jumped out of the Y-maze stage before the test started, while none of the control mice exhibited such behavior. In the Light/Dark transition test, the latency before entering into the light chamber was significantly decreased (Fig. 2B), while the number of transitions was significantly increased, suggesting a reduced attention and/or enhanced impulsivity.
We carried out microdialysis analysis in the prefrontal cortex of the cerebrum to directly examine the monoamine levels in the KO brain, because it is well documented that prefrontal cortical noradrenaline (NA) as well as dopamine (DA) plays an important role in spatial working memory. We found that the recovery of the NA level was significantly delayed compared with the DA level. It is reported that activation of the NA neurons of the locus coeruleus (LC) takes place in accord with the cognitive shifts that facilitate dynamic reorganization of target neural networks, permitting rapid behavioral adaptation to the demands of changing environmental imperatives3, indicating that all the behavioral defects of the Sirh11/Zcchc16 KO mice are somehow related to a dysregulation of the noradrenergic system in the brain. It also suggests that human SIRH11/ZCCHC16 may be a good candidate for X-linked intellectual disability (XLID), attention deficit/hyperactivity disorder (ADHD) and/or other diseases, such as autism. Therefore, it is probable that SIRH11/ZCCHC16 confers a critically important advantage in both the competition that occurs in daily life and eutherian brain evolution.
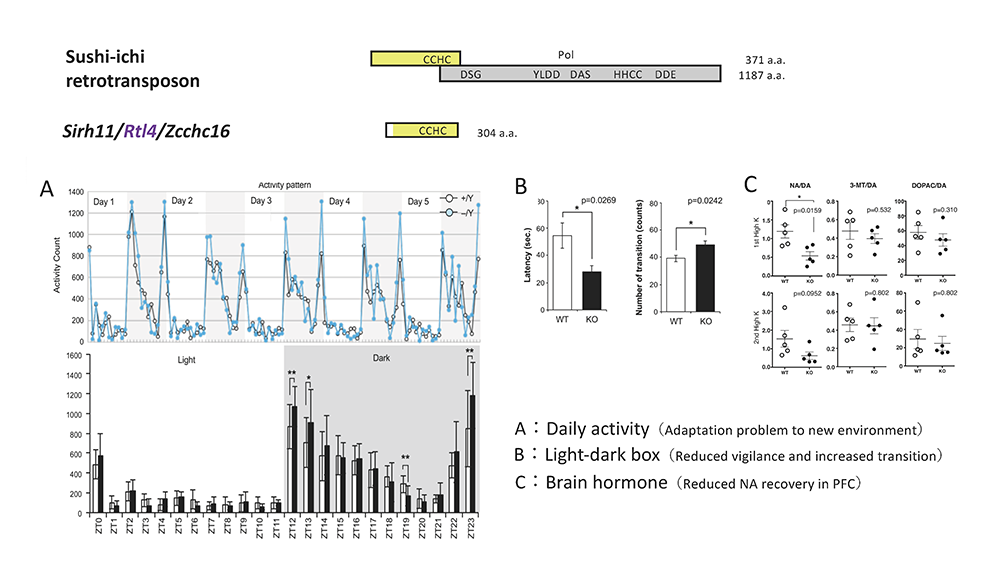
A: Home-cage activity test. (Top) The activity counts every hour over 5 days. The white and grey areas indicate the light and dark phases, respectively. (Bottom) The white and black bars represent the activity counts in the WT and KO mice, respectively. Zeitgeiber time (ZT) is shown on the x-axis. B: Light/Dark transition test. The left panel shows that KO mice exhibit a shorter latency time before entering into the light chamber. The right panel shows that the number of transitions is increased in KO mice. The white and black bars represent the WT and KO mice, respectively. C: Microdialysis analysis in the prefrontal cortex in the cerebrum. The recovery levels of DA and NA are shown after a perfusion of high potassium-containing artificial cerebrospinal fluid.
Original reference (Theme 4 reference 38)
- Irie M et al. Cognitive function related to the Sirh11/Zcchc16 gene acquired from an LTR retrotransposon in eutherians. PLoS Genet 11(9):e1005521 (2015).
Reference
- Bouret S and Sara S J. Network reset: A simplified overarching theory of locus coeruleus noradrenaline function. Trends Neurosci. 28, 574–582 (2005).
(2) Diversification of eutherian lineages by variations in SIRH11/ZCCHC16
Despites the significance of SIRH11/ZCCHC16 to eutherian brain evolution, the genomic record tells us that although it was exapted in the common eutherian ancestor (Fig. 3), it became a pseudogene in the Xenarthra lineage, such as armadillos and sloths, by a multiple frameshift events and nonsense mutations38 (Fig. 4, red stars indicating nonsense mutations). There is only one common nonsense mutation between these two animals in the C-terminus just before the CCHC RNA-binding domain (Fig. 4, blue stars surrounding a dotted red line), suggesting that loss of the CCHC domain led to functional inactivation of the SIRH11/ZCCHC16 protein followed by an independent accumulation of a large number of additional mutations.
Furthermore, each of two large variations in the SIRH11/ZCCHC16 ORF are found in several eutherian lineages, such as a lack of a CCHC RNA-binding domain in the C-terminus in gibbons and megabats, and a lack of the N-terminal half in New World monkeys and species belonging to New World and African rodents as well as groups of whales and cows (Fig. 5).
In collaboration with Akihiko Koga of the Primate Research Institute, Kyoto University, we found that all 6 species of the New World monkeys examined have a common nonsense mutation just after the start codon in the N-terminus, suggesting that they have a shorter SIRH11/ZCCHC16 ORF (approximately half size), starting from the second ATG. Among the hominoidea, three out of four genera of gibbons have lost normal SIRH11/ZCCHC16 function by either the deletion or the lack of the CCHC RNA-binding domain, while other members belonging to the hominids have a complete SIRH11/ZCCHC16 ORF. From the viewpoint of primate evolution, it is proposed that the common ancestor emigrated from Africa and somehow immigrated into South America ∼34 MYA4,5, possibly by an incidental current drift from Africa to South America that existed at that time4. Our data indicates a common ancestor of the New World monkeys in South America already had the mutation(s) leading to the N-terminal deletion. It is proposed that the currently living primates last shared a common ancestor 71–63 MYA and Asia was the ancestral home of the primates, although this is still controversial. Concerning the hominoids, it is suggested that the ancestor of African apes and humans entered Africa, while the gibbons (hylobatids) remained in Asia6. It is of interest to determine whether the loss of SIRH11/ZCCHC16 and/or change in its function did conferred selective advantages on the gibbons and New world monkeys. Our results show that SIRH11/ZCCHC16 may have contributed to the diversification of eutherians by lineage-specific structural changes after its domestication in the common eutherian ancestor, followed by putative species-specific functional changes that enhanced fitness, changes that occurred as a consequence of complex natural selection events.
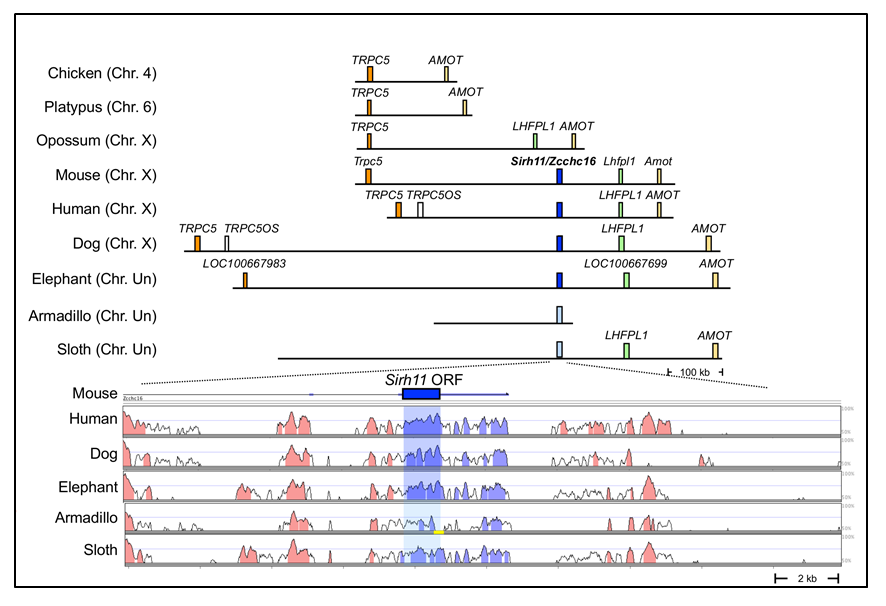
(Upper part) SIRH11/ZCCHC16 is conserved in an orthologous chromosomal region in eutherian mammals but is absent from the chicken (birds), platypus (monotremes) and opossum (marsupials). The blue boxes represent orthologous SIRH11/ZCCHC16 genes and the light blue boxes represent psuedoSIRH11/ZCCHC16 genes in two Xenarthran species, armadillos and sloths. (Lower part) A comparison of SIRH11/ZCCHC16 and its flanking genome sequences with 50~100% homology to the mouse genome in several eutherian mammals. The purple (SIRH11/ZCCHC16) and red (others) areas indicate evolutionarily conserved sequences, indicating reduced homology in armadillos and sloths.
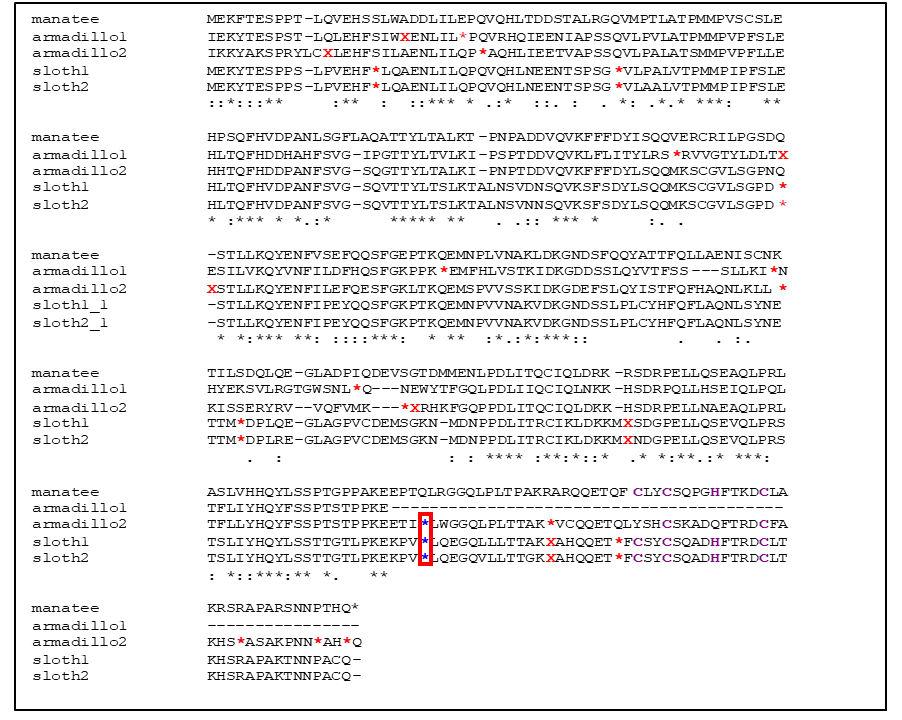
The armadillo and sloth species are compared with Florida manatee (an Afrotherian species). The red asterisks and red Xs show the sites of ORF termination and frameshift, respectively. The blue asterisks in a red box indicate a common mutation among three species. Purple characters indicate CCHC amino acids in the RNA binding domain.
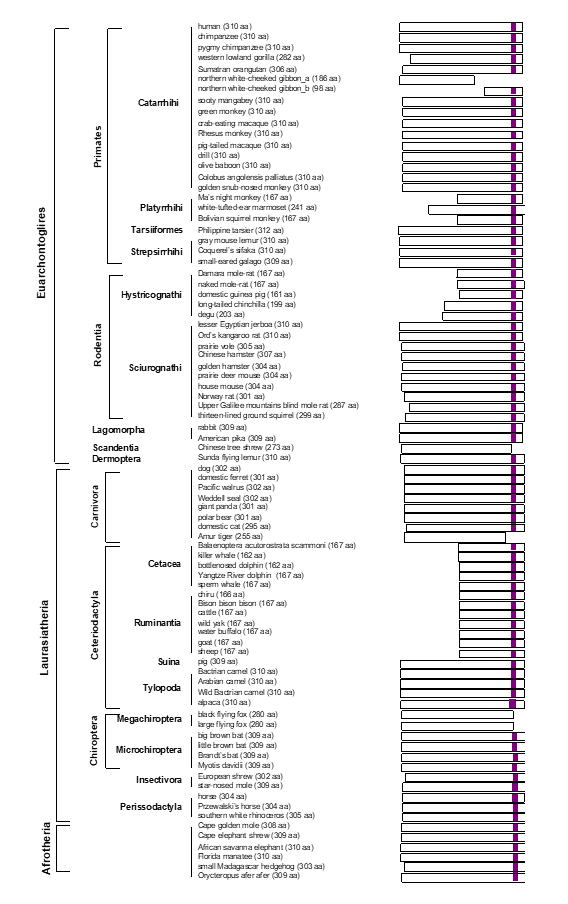
Two types of large deletions in the N-terminus and C-terminus including the CCHC RNA-binding domain are observed in several eutherian species. The purple boxes indicate the CCHC RNA-binding domain.
Original reference
- Irie M, Koga A, Kaneko-Ishino T* and Ishino F*. An LTR retrotransposon-derived gene displays lineage-specific structural and putative species-specific functional variations in eutherians. Front Chem 4:26 (2016).
References
- Houle A. The origin of platyrrhines: an evaluation of the Antarctic scenario and the floating island model. Am J Phys Anthropol 109, 541–559 (1999).
- Poux C, Chevret P, Huchon D, de Jong W W and Douzery E J P. Arrival and diversification of caviomorph rodents and platyrrhine primates in South America Syst Biol 55, 228–244 (2006).
- Springer M S, Meredith R W, Gatesy J, Emerling C A, Park J, Rabosky D L, Stadler T, Steiner C, Ryder O A, Janečka J E, Fisher C A and Murphy W J. Macroevolutionary dynamics and historical biogeography of primate diversification inferred from a species supermatrix. PLoS ONE 7:e49521 (2012).
3. Why are SIRH/RTL genes enriched on the X chromosome?
Among the 11 SIRH/RTL genes, PEG10, PEG11/RTL1 and SIRH3/LDOC1L/RTL6 are autosomal while another 8 are X-linked genes. Is there any reason and/or advantage for the acquisition of the LTR retrotransposon/retrovirus-derived genes on the X chromosome? Exaptation of LTR retrotransposons/retroviruses seems likely to be a very rare event because insertion of such sequences or their derivatives are typically harmful rather than advantageous. In addition, such insertions must occur in germ cells, such as sperms and eggs. It is reported that Ebola virus remains in the testes in surviving patients for a long time (WHO reports, 2017) and that koala retrovirus is transmitted vertically in surviving koala lineages like endogenous genes7, indicating that the insertion of certain viruses into the germline do occur, even if in very rare cases, followed by the unfortunate death of a large number of infected individuals. Insertions should occur randomly in autosomes and the sex chromosomes, X and Y. We propose that X chromosome inactivation (X-inactivation), a representative mammalian-specific epigenetic mechanism along with genomic imprinting, explains the biased X chromosome location of the SIRH/RTL genes.
In females, X-linked genes are typically subjected to random X-inactivation for gene dosage modulation, resulting in monoallelic expression. As a result, most SIRH/RTL genes exhibit monoallelic expression similar to the imprinted PEG10 and PEG11/RTL1 genes, which exhibit paternal-specific monoallelic expression (Themes 4, 5). It is reasonable to hypothesize that the integrated LTR retrotransposons/retroviruses or their derivatives would be present on only one of two homologous chromosomes at first, and that they would be harmful and behave like dominant negative genes (indicated as red bars).
- In the case of autosomal integration, both males and females would be considerably affected and would undergo lethality by their dominant negative effects (Fig. 6A). If the insertions were repressed by heavy DNA methylation, such individuals may have survived and the inserted DNAs would become fixed in the population according to the mechanism of genetic drift of neutral mutations. However, without selective pressure, most would lose function and became part of the “junk DNA” of genomes as is commonly observed in the current human genome.
- In the case of X chromosomal integration, males with an inserted X (indicated as X) would suffer lethality. Most females (XX) would also be thus affected, but some XX females would have a chance to survive because of X-inactivation (Fig. 6B).
- Emergence of XX females by X-inactivation
Some XX females that could silence such insertions and thus render them harmless by X-inactivation would be able to survive. Because females would have a mixture of two types of the cells in terms of X-inactivation, the viability of individuals would depend on which portions of the somatic cells were rescued by random X-inactivation, and the impact of the insertions would became less harmful on the whole. - A period of low penetrance of XX females
As the effect in all the XY males would be lethal, the viable XX females would always mate with wild type males. Therefore, the same scheme as shown Fig. 6B would be repeated (Fig. 6C). As paradoxical as it may seem, it may be critically important that all the mutant XY males die and only the normal wild-type males survive. The mutant XX females would thus always mate with normal healthy males and produce viable XX female offspring. In this scenario, even harmful DNA sequences could be maintained in a population at a low rate and thus be transmitted via heterogenous XX females from generation to generation. - A slight increase in XX females by the combined effects of mutations and X-inactivation
If the mutations occurred in the inserts were to make them less harmful, the survival rate of the XX females would be increased even if all the XY males were still inviable. - Emergence of XY males leading to expansion XX females
If further mutations in the inserts occurred in the germline in XX females (X to X, where X represents a viable type of X with the mutated insertion), they could be rendered harmless enough for the survival of some XY males, while XX females would also be increased because they would be viable without any need of X-inactivation, leading ultimately leading to a higher penetrance of X in the population (Fig. 6D). - Fixation of X in population
Once viable males with a slightly advantageous X appeared, this X would be propagated rapidly in both XY males and XX females (Fig. 6D, bottom right) and the inserts would finally become fixed in the population and behave as endogenous genes.
The X-linked inserts are always repressed in one half of the somatic cells in each individual, and thus would have a chance of being transmitted to the next generation even if they were to have a harmful nature. The X-linked genes are always active in another half of the somatic cells in each individual, so there would be a chance of being selected if advantageous mutations were to occur. We assume that the X chromosomes may have provided an environment for a “peaceful coexistence” with certain harmful foreign inserts.
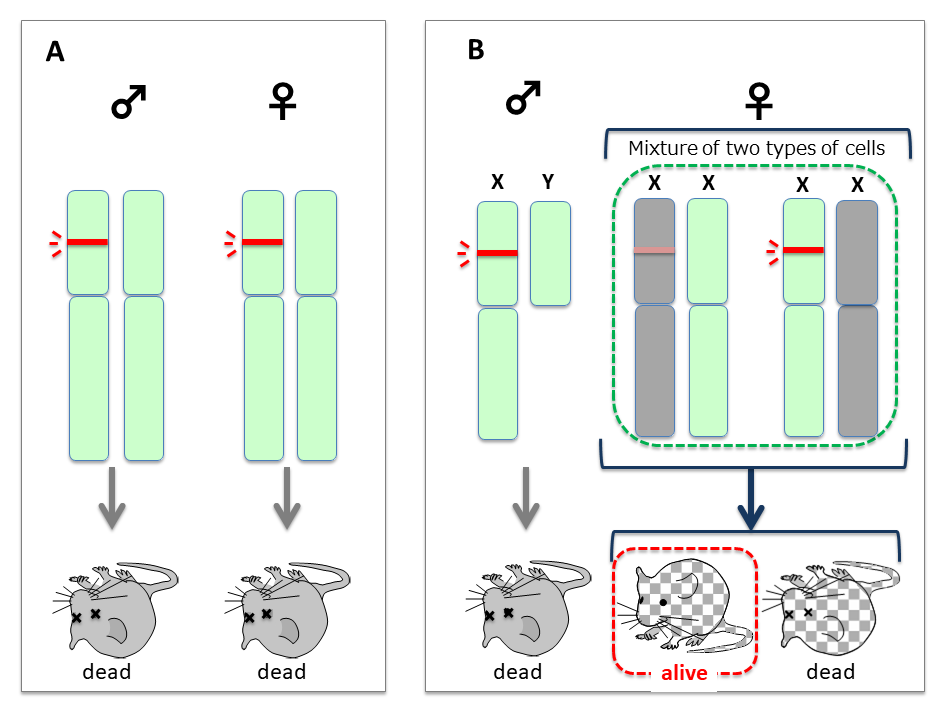
Comparison of the results of retrotransposon/retrovirus integration in an autosome (A) and the X chromosome (B). Harmful effects of the insert (red bars) would be reduced by X-inactivation (shaded). According to the nearly neutral theory of molecular evolution, a less harmful mutation can be fixed in a population by a random drift mechanism. (C) The insert would be maintained by transmission from heterogenous XX mutant females in each generation.(D)If a viable type of X with the mutated insertion (blue) emerged, XY males would appear and XX females would become viable without any need of X-inactivation. Homo XX females would then appear in the population (bottom right).
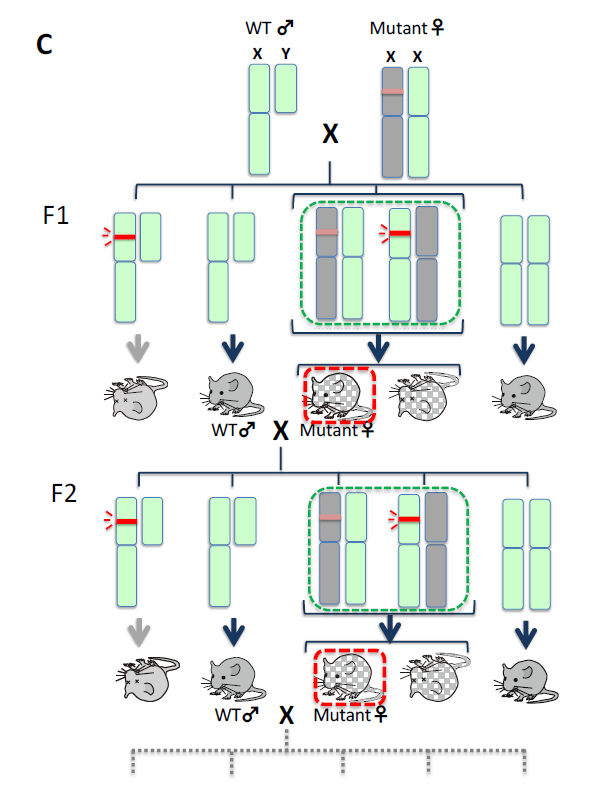
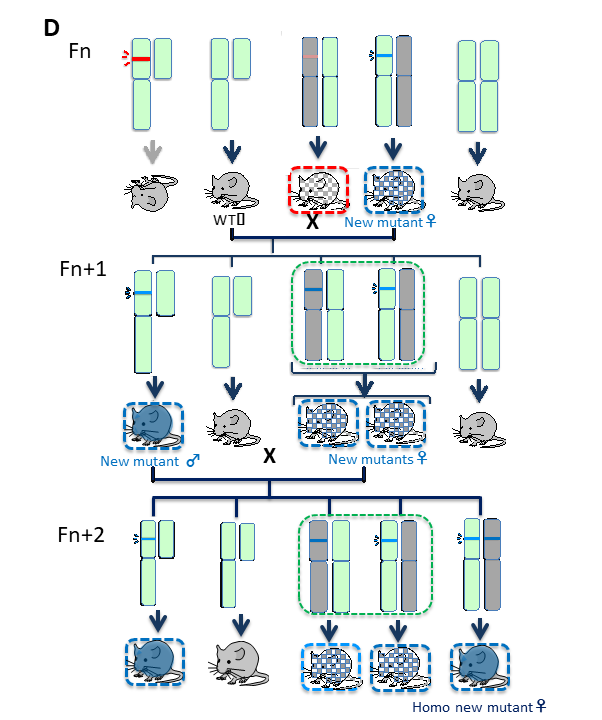
Original reference
- Kaneko-Ishino T, Irie M and Ishino F. Mammalian-specific traits generated by LTR retrotransposon-derived SIRH genes. In Evolutionary Biology: Self/NonSelf Evolution, Species and Complex Traits Evolution, Methods and Concepts (ed. Pontarotti P), Springer International Publishing, pp.129-145 (2017).
Reference
- Tarlinton R E, Meers Joanne and Young P R. Retroviral invasion of the koala genome. Nature 442, 79-81 (2006).
4. Mammalian evolution by genes exapted from LTR retrotransposons and retroviruses
Comprehensive genome analysis has demonstrated that all the SIRH/RTL genes exhibit the highest homology to the suchi-ichi retrotransposon (Themes 4 and 5). LTR retrotransposons and retroviruses have very similar structures, although only the latter have the Env gene for infection (Fig. 7). Therefore, it is probable that the origin of SIRH/RTL genes was a certain retrovirus very similar to the suchi-ichi retrotransposon present at the time (perhaps extinct thereafter). In the human genome, the protein coding genes occupy only 1.5 % of the total, while retrotransposons occupy approximately 40 % (Fig. 8). Therefore, retrotransposons have long been thought to be useless “junk.” However, our research clearly demonstrates that SIRH/RTL genes playing essential roles in development and/or the brain in mammals were derived from LTR retrotransposons/endogenous retrotransposons (ERVs) occupying approximately 8 % of the human genome. Therefore, we propose the concept of “mammalian-specific exapted genes”, each of which functions in a mammalian-specific manner, as the description suggests.
The idea that host organisms make use of such transposable DNA elements as a genetic resource of genes for novel purposes has been formally proposed by Gould and his colleagues (e.g. Brosius and Gould, PNAS 1992). There are several important examples, including: telomerase in eukaryotes from LTR retrotransposon or retrovirus8, recombination activating genes (RAG1 and RAG2) for immunoglobulin V(D)J recombination in vertebrates from a DNA transposon9,10, transcription factors essential for the light response in plants, far-red elongated hypocotyls 3 (FHY3) and far-red-impaired response (FAR1) genes from DNA transposons11-13. Thus, it is clear that the exaptation of such transposable elements has exerted a profound effect on quite a large number of animals and plants during the course of biological evolution, even if only few cases are currently known.
Eleven SIRH/RTL genes and more than 15 paraneoplastic Ma-antigen (PNMA) genes from another LTR retrotransposon were exapted around the time of the establishment of the therian and eutherian mammals as well as later during the radiation of mammals, when several SYNCYTINs14-16 and FEMATRIN17 genes that are active in the cell fusion process in the placenta were exapted from Env genes from different retroviruses in a lineage-specific manner (Fig. 9). Why do mammals have so many exapted genes? We assume that this may be because that the elucidation of the genome and its functional analyses in mammals preceded such work in the vertebrates and other organisms. We predict that many more examples will be discovered in a wide variety of organisms in the near future and also that the gene exaptation from foreign DNA is an important driving force in biological evolution.
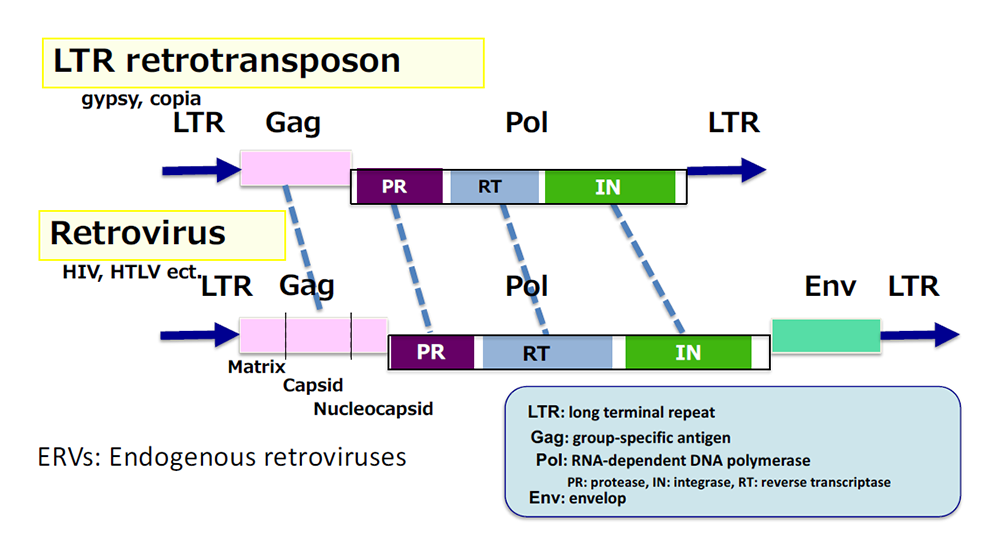
They both have Gag and Pol genes for self-amplification but only retroviruses have an Env gene, an envelope gene, which is necessary for infecting other cells. Retroviral DNA integrated in the genome are called endogenous retrovirus (ERV).
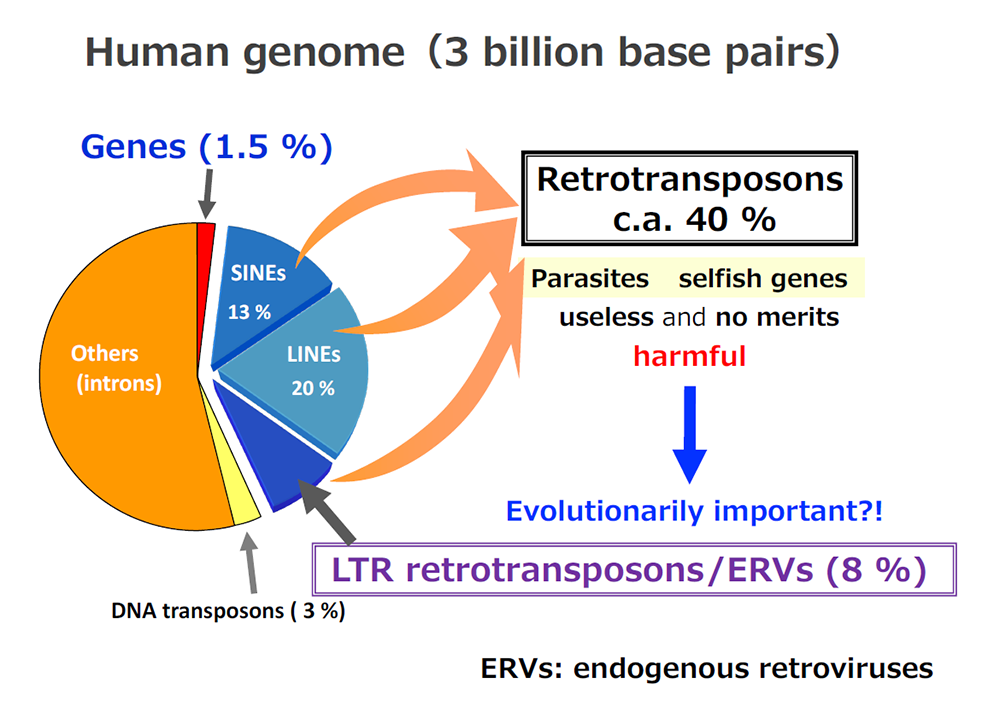
Approximately 40 % of the human genome is occupied by retrotransposons composed of long interspersed nuclear elements (LINEs, 20 %), short interspersed nuclear elements (SINEs, 13 %) and LTR retrotransposons/ERVs (8%).
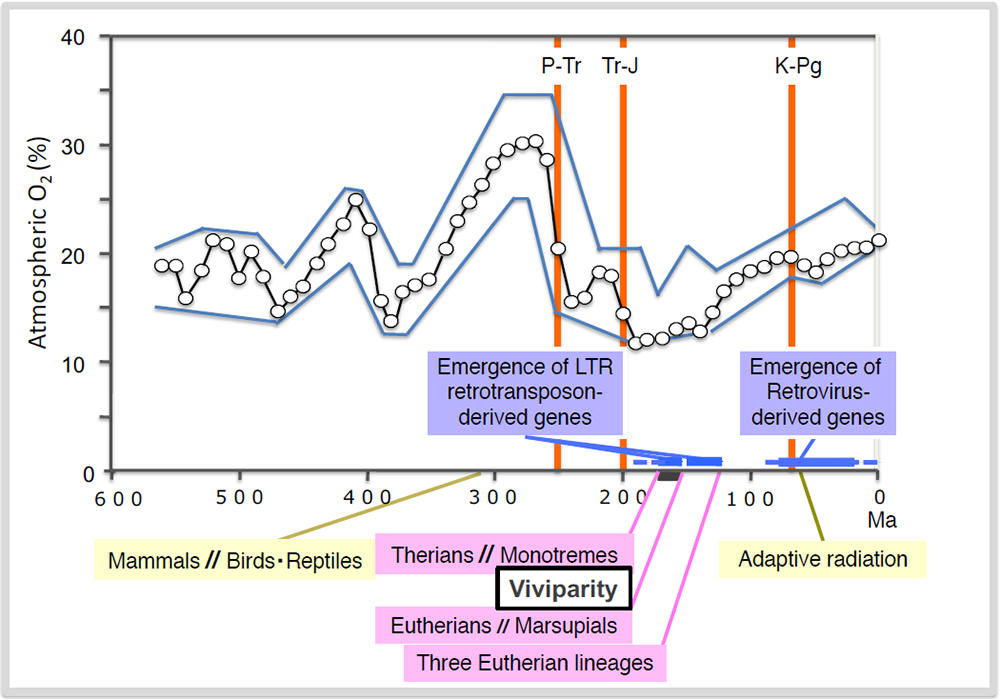
Exaptation of SIRH/RTL genes and the emergence of viviparity occurred under the lowest atmospheric oxygen concentration in the history of life on Earth that occurred between the P-Tr and Tr-J boundaries. Exaptation of retrovirus-derived SYNCYTIN genes occurred after the K-Pg boundary and associated with the extinction of the dinosaurs. Three out of the five mass extinction events related to mammalian history are indicated as orange lines. Permian-Triassic; P-Tr, Triassic-Jurassic; Tr-J and Kreide (Cretaceous)-Paleogene; K-Pg boundaries, respectively.
Original reference
- Kaneko-Ishino T and Ishino F. The role of genes domesticated from LTR retrotransposons and retroviruses in mammals. Front Microbiol 3, 262 (2012).
References
- Nakamura T M and Cech T R. Reversing time: origin of telomerase. Cell 92, 587–590 (1998).
- Agrawal A, Eastman Q M and Schatz D G. Transposition mediated by RAG1 and RAG2 and its implications for the evolution of the immune system. Nature 394,744–751 (1998).
- Hiom K, Mele M and Gellert M. DNA transposition by the RAG1 and RAG2 proteins: a possible source of oncogenic translocations. Cell 94, 463–470 (1998).
- Lisch D R, Freeling M, Langham R J and Choy M Y. Mutator transposase is widespread in the grasses. Plant Physiol 125,1293–1303 (2001).
- Hudson M E, Lisch D R and Quail P H. The FHY3 and FAR1 genes encode transposaserelated proteins involved in regulation of gene expression by the phytochrome A-signaling pathway. Plant J 34, 453–471 (2003).
- Lin R, Ding L, Casola C, Ripoll D R, Feschotte C and Wang H. Transposase-derived transcription factors regulate light signaling in Arabidopsis. Science 318, 302–1305 (2007).
- Mi S, Lee X, Li X-P, Veldman G M, Finnerty H, Racie L, LaVallie E, Tang X-Y, Edouard P, Howes S, Keith J C Jr and McCoy J M. Syncytin is a captive retroviral envelope protein involved in human placental morphogenesis. Nature 403, 785–789 (2000).
- Dupressoir A, Vernochet C, Bawa O, Harper F, Pierron G, Opolon P and Heidmann T. Syncytin-A knockout mice demonstrate the critical role in placentation of a fusogenic, endogenous retrovirusderived, envelope gene. Proc Natl Acad Sci USA 106, 12127–12132 (2009).
- Lavialle C, Cornelis G, Dupressoir A, Esnault C, Heidmann O, Vernochet C and Heidmann T. Paleovirology of ‘syncytins’, retroviral env genes exapted for a role in placentation. Philos Trans R Soc Lond B Biol Sci 368, 20120507 (2013).
- Nakaya Y, Koshi K, Nakagawa S, Hashizume K and Miyazawa T. Fematrin-1 is involved in fetomaternal cell-to-cell fusion in Bovinae placenta and has contributed to diversity of ruminant placentation. J Virol 87, 10563–10572 (2013).
5. What was the selective pressure on emergence of viviparity in mammals?: a relationship to oxygen concentration in the atmosphere
The genomic record shows that PEG10 is present only in therians (eutherians and marsupials) among the vertebrates, indicating that PEG10 emerged after the diversification of the therians from the monotremes that occurred 166 million years ago (MYA) and before the split of the marsupials and eutherians 148 MYA (Fig. 10). This event fits well with the emergence of viviparity in mammals (Figs. 9 and 10), and PEG10 has an essential function in placenta formation (Theme 4). Subsequently, PEG11/RTL1 and the remaining 9 SIRH/RTL genes emerged between the split of the therians (148 MYA) and that of the three major groups of eutherians (120 MYA), such as Boreotheria (including Euarchontoglires and Laurasiatheria), Afrotheria and Xenarthra) (Figs. 9 and 10), a split caused by the division of the supercontinent Pangea. Among them at least PEG11/RTL1 and SIRH7/LDOC1/RTL7 have essential placental functions and presumably contributed to the evolution of the eutherian type of viviparous reproduction (Theme 5) . All of these genes have been conserved in humans and mice after their exaptation, while some became pseudogenes in other eutherian lineages (see Spin-off 2).
According to the Darwinian theory of evolution, mutations and selective pressure are two important factors by which natural selection proceeds. What were the environmental conditions that promoted the mammalian viviparous reproductive system and/or exaptation of a series of SIRH/RTL genes? Berner et al. reported a relationship between the atmospheric oxygen concentration and biological evolution in the Phanerozoic eon (from 550 MYA to the present)18 (Fig. 9). According to their data, the highest atmospheric oxygen (30%) was observed in the Carboniferous period 300 MYA, however, it dramatically decreased to 15% around the extinction at the Permian-Triassic (P-Tr) boundary 250 MYA. After the next mass extinction at the Triassic-Jurassic (Tr-J) boundary approximately 200 MYA, the period with the lowest atmospheric oxygen (12–13%) appeared from 190 to 150 MYA. Therefore, the divergence of the therians from the monotremes (166 MYA), and also the eutherian and marsupial spilt (160 MYA), occurred under the condition of the lowest atmospheric oxygen. This suggest that the low oxygen concentration might be a major driving force in the establishment of the current viviparous reproductive system, although it might conceivably be a fortuitous coincidence. It is apparent that oviparous reproduction, with embryonic development within the egg shell, is directly affected by the atmospheric oxygen concentration because it is dependent on the ratio of the oxygen penetration through the egg shell. In contrast, in viviparous reproduction there is direct oxygen supply to embryos via the placental blood circulating system along with an effective nutrient supply, which would confer an advantage in such a severe situation.
The adaptive radiation of mammals took place after the extinction of the dinosaurs at the Kreide (Cretaceous)-Paleogene (K-Pg) boundary 65 MYA. At that time, the atmospheric oxygen concentration was gradually increased to a point near the present concentration (20%). It is highly probable that large vacant ecological niches on land, sea and sky allowed the ancestors of eutherian and marsupial lineages to become diversified by continuing the acquisition of retrovirus-derived genes, such as SYNCYTINs and FEMATRIN (Spin-off 4), suggesting that a release from a powerful selective pressure also serves as a major driving force in macroevolution.
Approximately 8 % of the human genome is occupied by LTR retrotransposons and ERVs (Spin-off 4). We predict that there remain a large number of unidentified genes exapted from these elements as primate-, simian-, hominids- and human-specific genes acquired in a step-by-step manner during the course of human evolution.
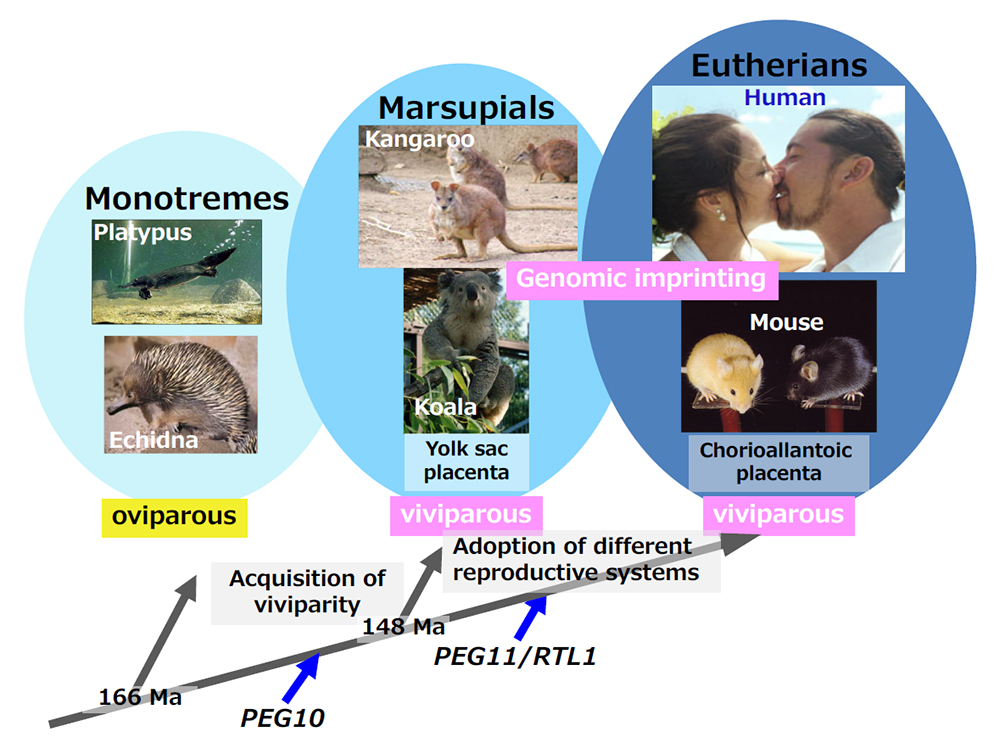
PEG10 emerged when mammals acquired a viviparous reproduction system (the therians) and PEG11/RTL1 emerged when the eutherians, having chorioallantoic placentas, appeared. Genomic imprinting is conserved in two viviparous mammalian groups, the marsupials and eutherians.
Original reference (Theme 6, reference 28)
- Kaneko-Ishino T and Ishino F. Mammalian-specific genomic functions: Newly acquired traits generated by genomic imprinting and LTR retrotransposon- derived genes in mammals. Proc Jpn Acad Ser B Phys Biol Sci 91, 511-538 (2015).
Reference
- Berner, R.A., Vandenbrooks, J.M. and Ward, P.D. Evolution. Oxygen and evolution. Science 316, 557–558 (2007).